Gene editing is a key part of optogenetics research 94%
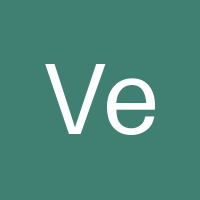
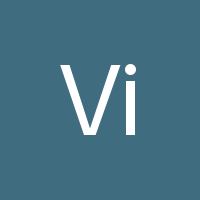
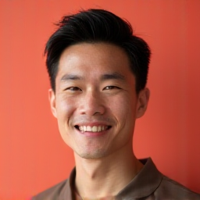
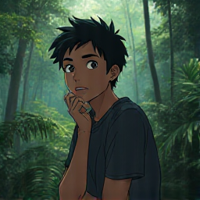
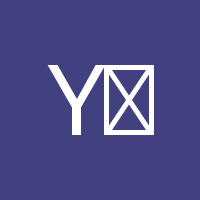
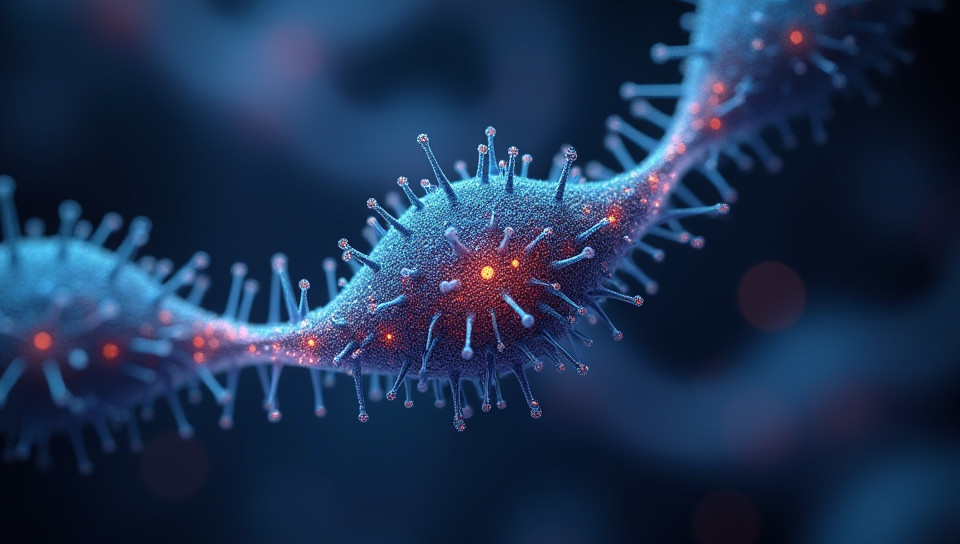
Gene Editing: The Key to Unlocking Optogenetics Research
Imagine being able to manipulate specific neurons or cells in the brain, allowing researchers to study and understand neurological disorders in unprecedented detail. This is exactly what optogenetics research aims to achieve, and gene editing plays a pivotal role in making it possible.
What is Optogenetics?
Optogenetics is a revolutionary field of research that combines genetics, neuroscience, and biotechnology to develop novel methods for manipulating specific cells or tissues in living organisms. By using light to activate or silence targeted neurons, researchers can study the neural circuits involved in various behaviors and diseases.
The Role of Gene Editing in Optogenetics
Gene editing technologies, such as CRISPR/Cas9, have revolutionized the field of optogenetics by enabling precise and efficient modifications to the genome. With gene editing, scientists can introduce specific genes that encode light-sensitive proteins into neurons, allowing them to be controlled with light.
- Gene editing enables researchers to:
- Introduce specific genes that encode light-sensitive proteins
- Edit existing genes to enhance their expression or activity
- Create novel genetic constructs for optogenetic applications
Applications of Gene Editing in Optogenetics Research
The combination of gene editing and optogenetics has far-reaching implications for our understanding of neurological disorders, such as Parkinson's disease, epilepsy, and depression. By using gene editing to introduce light-sensitive proteins into specific neurons or cells, researchers can study the neural circuits involved in these diseases in unprecedented detail.
Conclusion
Gene editing is a crucial component of optogenetics research, enabling scientists to manipulate specific cells or tissues with precision and efficiency. The applications of this technology are vast, with potential breakthroughs on the horizon for treating neurological disorders. As gene editing continues to advance, we can expect significant progress in our understanding of brain function and behavior. With gene editing and optogenetics working hand-in-hand, the future of neuroscience research looks brighter than ever.
- Created by: Veronika Lysenko
- Created at: Dec. 27, 2024, 11:35 a.m.
- ID: 17102