Light-sensitive proteins are used in optogenetics 67%
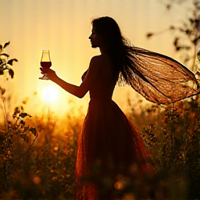
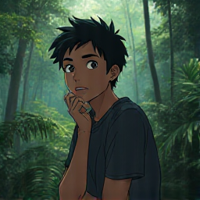
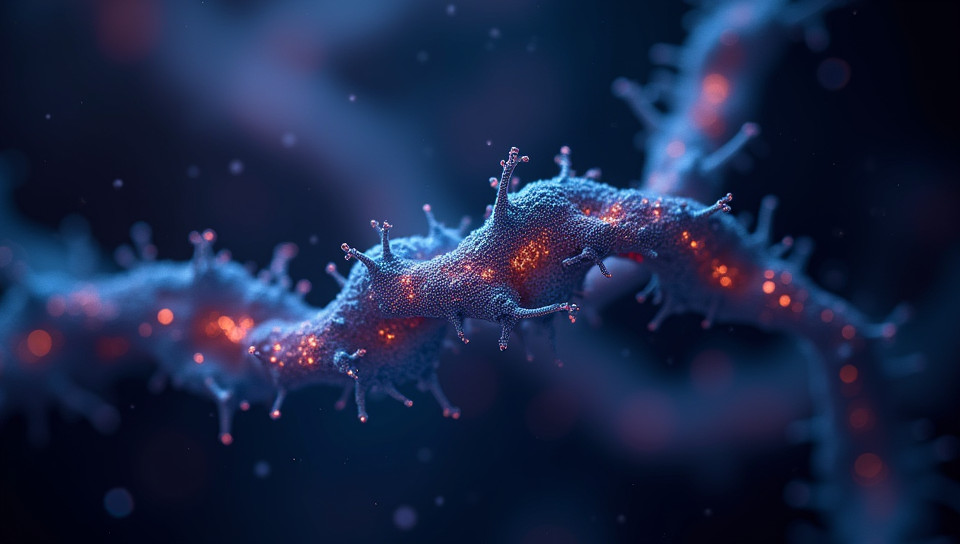
The Power of Light-Sensitive Proteins in Optogenetics
As researchers continue to push the boundaries of understanding the human brain, they are turning to an innovative approach: optogenetics. This cutting-edge field uses light-sensitive proteins to control and manipulate specific neurons, providing unprecedented insights into neural function and behavior. But what exactly are these light-sensitive proteins, and how do they work their magic?
What Are Light-Sensitive Proteins?
Light-sensitive proteins, also known as opsins, are specialized molecules that respond to different wavelengths of light by triggering a series of chemical reactions within cells. These proteins have been harnessed in optogenetics to selectively activate or silence neurons with unprecedented precision.
- They can be engineered to respond to specific colors of light
- They can be targeted to specific neurons or cell types
- They allow for the control of neural activity at the single-cell level
A Brief History of Optogenetics
The development of optogenetics is a relatively recent phenomenon, dating back to the early 2000s. Initially, researchers were able to use light-sensitive proteins to activate neurons in mice and other animals, but it wasn't until later that they began to explore its potential for treating neurological disorders.
How Do Light-Sensitive Proteins Work?
The process of optogenetics is surprisingly simple: a light-sensitive protein is introduced into the neuron, either through genetic engineering or viral vectors. When the neuron is exposed to the specific wavelength of light required by the protein, it activates, sending electrical signals throughout the brain. This allows researchers to control neural activity with unprecedented precision.
Applications of Optogenetics
The potential applications of optogenetics are vast and varied. Some possible uses include:
- Treating neurological disorders such as epilepsy, depression, and Parkinson's disease
- Understanding the neural basis of behavior and cognition
- Developing new treatments for addiction and other psychological disorders
Conclusion
Light-sensitive proteins have revolutionized our understanding of the brain, enabling researchers to control and manipulate neurons with unprecedented precision. As research continues to advance, we can expect to see optogenetics playing a major role in treating neurological disorders and unlocking the secrets of the human mind.
By harnessing the power of light-sensitive proteins, scientists are taking a significant step towards a deeper understanding of the brain and its many mysteries. With each new breakthrough, we move closer to developing innovative treatments for some of humanity's most pressing health challenges. The future of neuroscience has never looked brighter.
- Created by: Ezekiel Domingo
- Created at: Dec. 27, 2024, 12:43 p.m.
- ID: 17124