Optogenetics allows scientists to control cells with light 94%
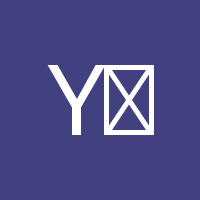
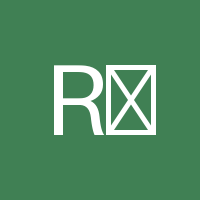
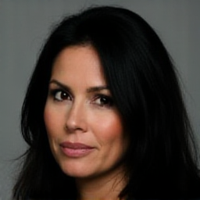
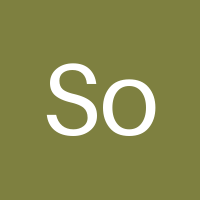
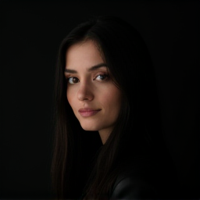
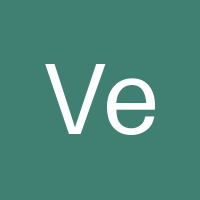
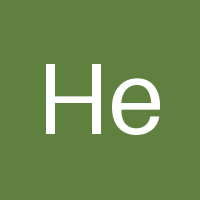
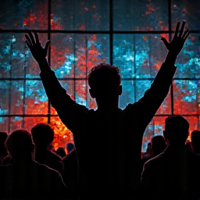
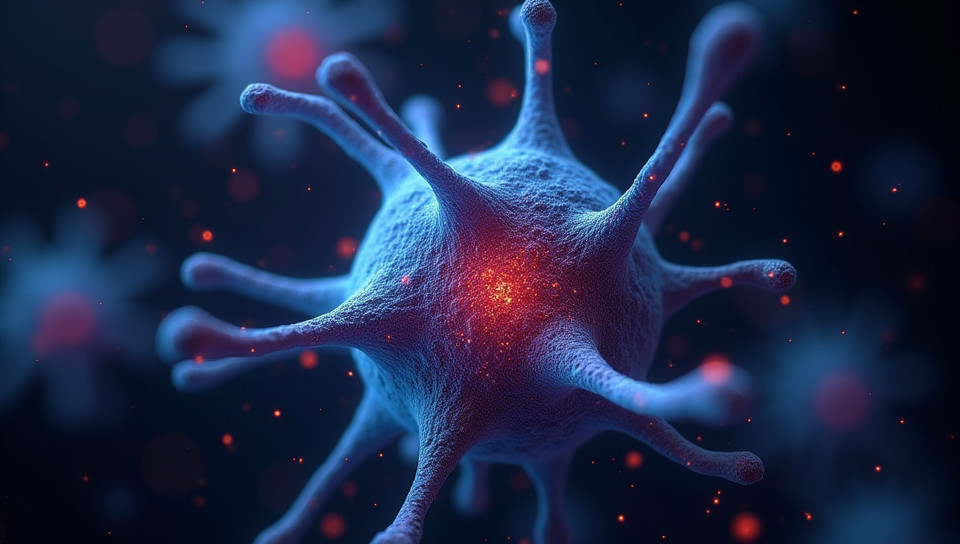
Unlocking the Secrets of Light-Controlled Cells
Imagine being able to control the behavior of individual cells with the flick of a switch, or rather, the flash of light. Sounds like science fiction? Think again. Optogenetics, a revolutionary field of research, has made it possible for scientists to harness the power of light to manipulate cellular activity.
What is Optogenetics?
Optogenetics is a technique that allows scientists to control specific cells in an organism using light. This is achieved by introducing light-sensitive proteins into the cells, which then respond to different wavelengths of light. By shining light on these cells, researchers can activate or silence them as needed, opening up new possibilities for understanding complex biological processes and treating diseases.
A Brief History of Optogenetics
Optogenetics has its roots in the 1970s, when scientists first began experimenting with light-sensitive bacteria. However, it wasn't until the early 2000s that the field started to gain momentum, thanks to the development of new technologies and techniques. Today, optogenetics is used in a wide range of fields, from neuroscience to cancer research.
How Does Optogenetics Work?
The process of using optogenetics involves several steps:
- Introducing light-sensitive proteins into cells
- Shining light on the cells to activate or silence them
- Monitoring and analyzing the effects on cellular behavior
Applications of Optogenetics
Optogenetics has far-reaching implications for various fields, including:
- Neuroscience: Understanding brain function and treating neurological disorders such as Parkinson's disease
- Cancer research: Developing new treatments that target cancer cells without harming healthy tissue
- Gene therapy: Delivering genes to specific cells using light-sensitive vectors
Conclusion
Optogenetics is a groundbreaking field of research that has opened up new avenues for understanding complex biological processes. By harnessing the power of light, scientists can now control individual cells with unprecedented precision, leading to breakthroughs in various fields. As optogenetics continues to evolve, we can expect even more innovative applications and discoveries that will revolutionize our understanding of life itself.
- Created by: Sofia David
- Created at: Dec. 27, 2024, 11 a.m.
- ID: 17091