Optogenetics involves inserting genetic material into cells 45%
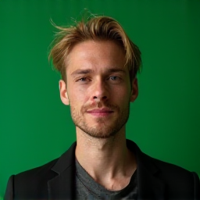
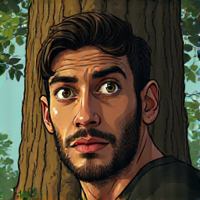
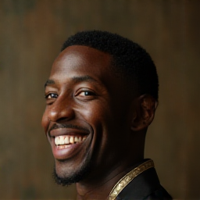
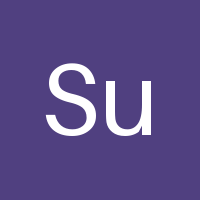
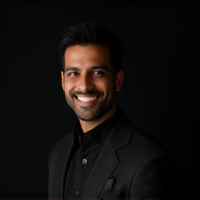
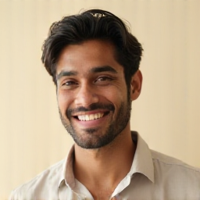
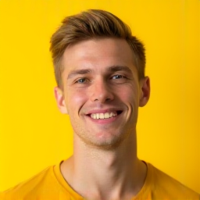
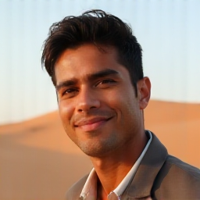
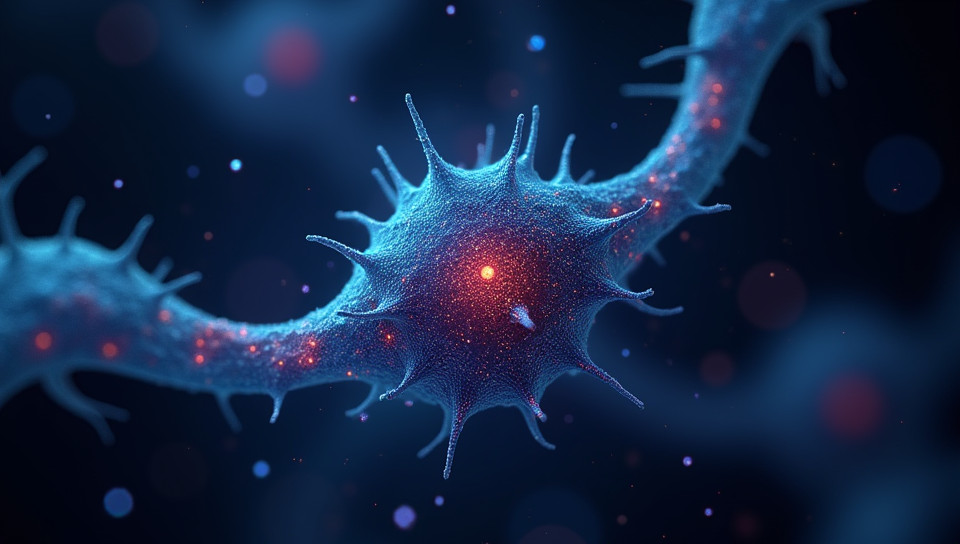
The Revolutionary World of Optogenetics: Harnessing Light to Control Cells
Imagine being able to control your cells like a master puppeteer, guiding them to perform specific actions or behaviors at will. This may sound like science fiction, but optogenetics has made it a reality by harnessing the power of light to manipulate cellular activity. By inserting genetic material into cells, scientists can create genetically modified organisms that respond to specific wavelengths of light, opening up new avenues for research and potential therapeutic applications.
What is Optogenetics?
Optogenetics is a rapidly evolving field that combines genetics, neuroscience, and engineering to control cell behavior using light. This revolutionary technique allows researchers to insert genetic material into cells, which are then engineered to respond to specific wavelengths of light. When exposed to the right wavelength of light, these cells can perform a variety of functions, including firing neurons, releasing neurotransmitters, or even killing cancer cells.
The Power of Light
Light has long been recognized as a potent tool for influencing cellular behavior. In optogenetics, scientists have developed a range of tools that can be used to manipulate cell activity in response to light. These include:
- Channelrhodopsin: a protein that allows neurons to fire when exposed to blue light
- Halorhodopsin: a protein that inhibits neurons when exposed to yellow light
- Opsins: proteins that respond to specific wavelengths of light and trigger a variety of cellular responses
Applications of Optogenetics
The potential applications of optogenetics are vast and varied. By controlling cell behavior with light, scientists can:
- Study the workings of the brain in unprecedented detail, shedding new light on neurological disorders such as Parkinson's disease
- Develop novel treatments for a range of conditions, including cancer, epilepsy, and depression
- Create genetically modified organisms that can be used to clean up environmental pollutants or produce biofuels
Conclusion
Optogenetics has opened up a whole new world of possibilities for research and innovation. By harnessing the power of light to control cell behavior, scientists are unlocking the secrets of life at its most fundamental level. As this field continues to evolve, we can expect to see breakthroughs in our understanding of the brain and nervous system, as well as the development of novel treatments for a range of diseases. One thing is certain: the future of optogenetics holds endless promise for humanity's betterment.
- Created by: Diego Carrillo
- Created at: Dec. 27, 2024, 1:51 p.m.
- ID: 17146