Precise neural control is the goal of optogenetics 98%
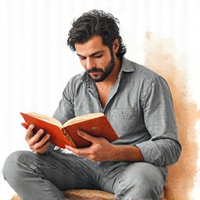
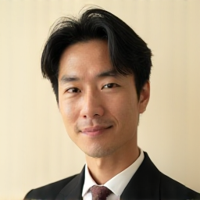
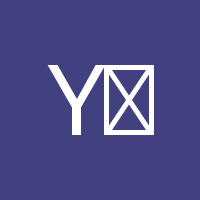
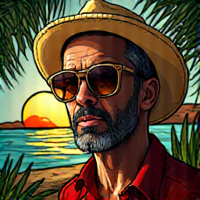
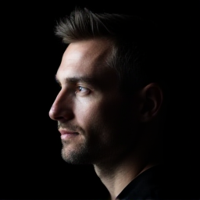
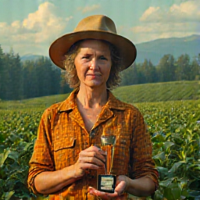
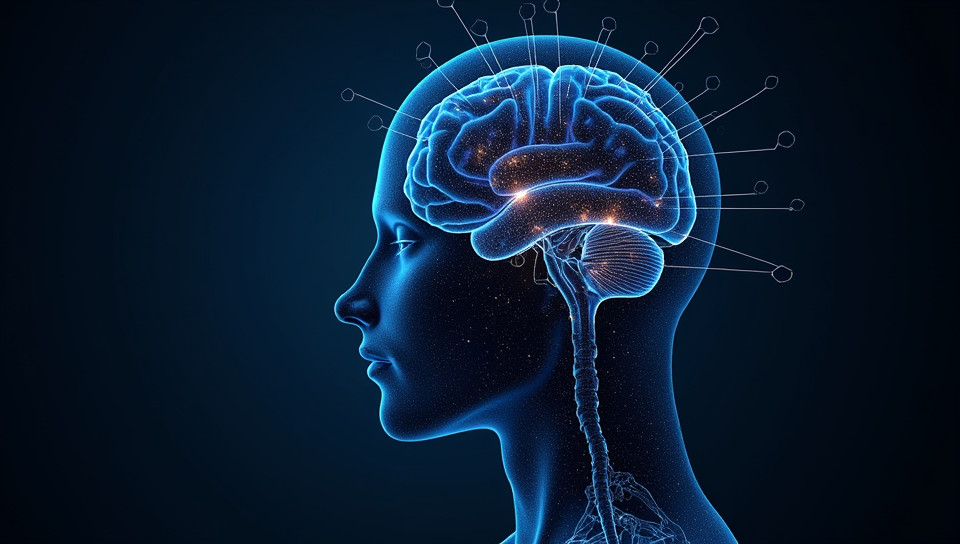
The Future of Neuroscience: Unlocking Precise Neural Control with Optogenetics
Imagine being able to control specific neurons in the brain, turning them on or off at will, without causing harm to surrounding cells. This is the promise of optogenetics, a revolutionary field that combines genetics and optics to manipulate neural activity with unprecedented precision. As researchers continue to push the boundaries of this technology, we may soon be able to treat a wide range of neurological disorders, from depression and anxiety to Parkinson's disease and epilepsy.
What is Optogenetics?
Optogenetics is a technique that uses light-sensitive proteins, such as channelrhodopsin or halorhodopsin, to control neural activity. By injecting these proteins into specific neurons, scientists can activate or inhibit them using different wavelengths of light. This allows for precise manipulation of brain function, enabling researchers to study complex neurological processes and develop new treatments.
How Does Optogenetics Work?
The process of optogenetics involves several key steps:
- Delivering the light-sensitive protein to the target neuron
- Activating or inhibiting the protein using a specific wavelength of light
- Measuring the resulting changes in neural activity
This is typically done using a combination of genetics, neuroscience, and engineering. The genetic component involves introducing the light-sensitive protein into the brain, while the neurological component requires understanding the complex interactions between neurons.
Applications of Optogenetics
The potential applications of optogenetics are vast and varied:
- Treating neurological disorders: By selectively activating or inhibiting specific neurons, researchers may be able to develop new treatments for a wide range of conditions.
- Studying brain function: Optogenetics allows scientists to manipulate neural activity in real-time, enabling a deeper understanding of complex brain processes.
- Developing novel therapies: The precision and control offered by optogenetics make it an attractive option for developing new treatments that target specific neurons or pathways.
Conclusion
Precise neural control is the goal of optogenetics, and this technology holds immense promise for advancing our understanding of the brain and treating neurological disorders. As researchers continue to refine and expand upon this technique, we may soon be able to unlock the secrets of the human mind and develop new treatments that improve the lives of millions. The future of neuroscience is bright, and optogenetics is leading the way.
- Created by: Miguel Ángel Acosta
- Created at: Dec. 27, 2024, 3 p.m.
- ID: 17168