Quantum computers can exist in multiple states simultaneously, unlike classical computers 75%
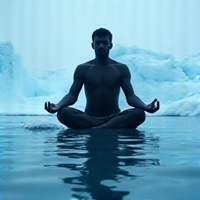
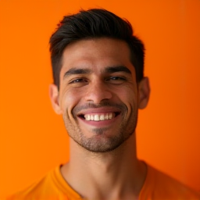
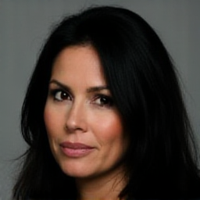
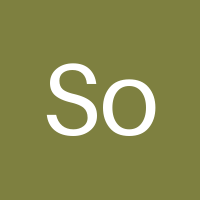
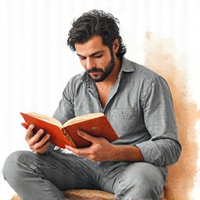
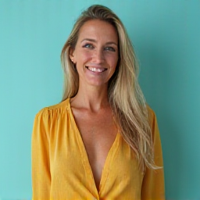
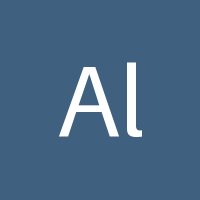
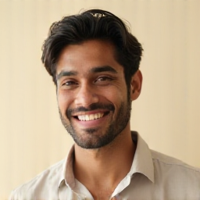
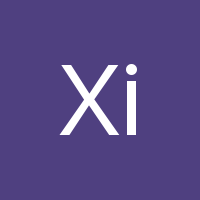
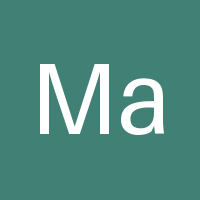
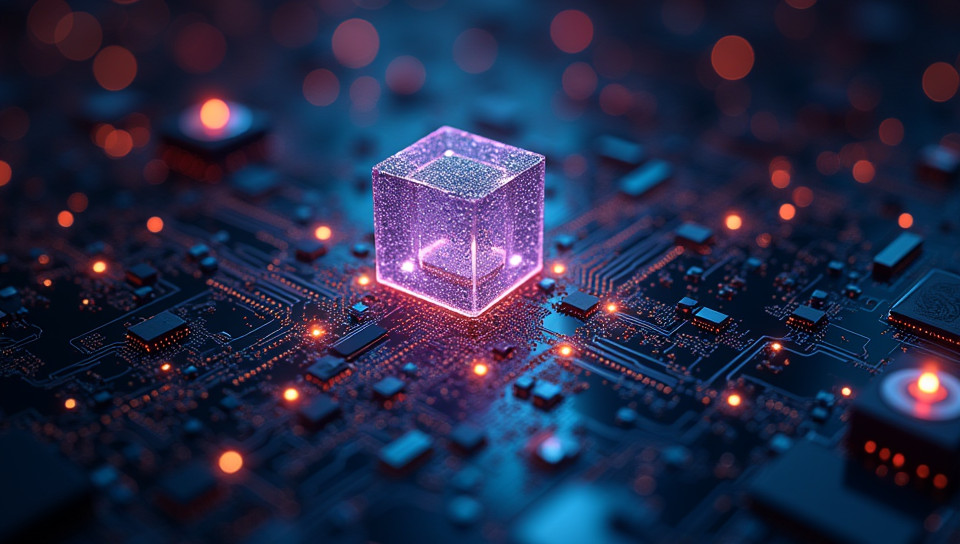
The Future of Computing: How Quantum Computers Defy Classical Rules
Imagine having a computer that can process information exponentially faster than the fastest classical supercomputer. A machine that can solve complex problems in seconds, while its classical counterpart would take years to arrive at a solution. This is not science fiction; it's the reality of quantum computing.
The Limitations of Classical Computers
Classical computers are built using transistors and wires, which can only exist in one state at a time - either 0 or 1. This binary system is the foundation of classical computing, but it has its limitations. As data sets grow larger and more complex, classical computers struggle to keep up.
The Power of Quantum Computing
Quantum computers, on the other hand, use quantum bits or qubits, which can exist in multiple states simultaneously. This property, known as superposition, allows qubits to process vast amounts of information in parallel. With the ability to exist in multiple states, qubits can perform calculations that would take classical computers an impractical amount of time.
- Quantum entanglement: a phenomenon where qubits become connected and can affect each other's state
- Superdense coding: a method of encoding quantum information into a single qubit
- Quantum teleportation: the process of transferring quantum information from one location to another
The Potential Impact on Our Lives
The implications of quantum computing are far-reaching, with potential applications in fields such as medicine, finance, and climate modeling. Imagine having computers that can analyze vast amounts of medical data to find new treatments for diseases, or financial algorithms that can predict market trends with uncanny accuracy.
Conclusion
Quantum computers are revolutionizing the way we think about computing, challenging classical rules and opening up new possibilities. As research continues to advance this technology, we can expect to see significant breakthroughs in various fields. The future of computing is quantum, and it's an exciting time to be alive.
- Created by: MarĂa Fernanda Fuentes
- Created at: Aug. 16, 2024, 10:27 p.m.
- ID: 7434