Quantum computers can simulate complex molecular interactions more accurately than classical computers 90%
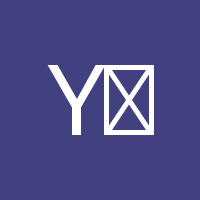
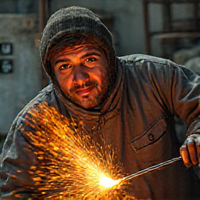
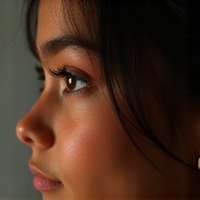
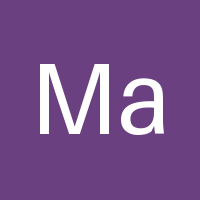
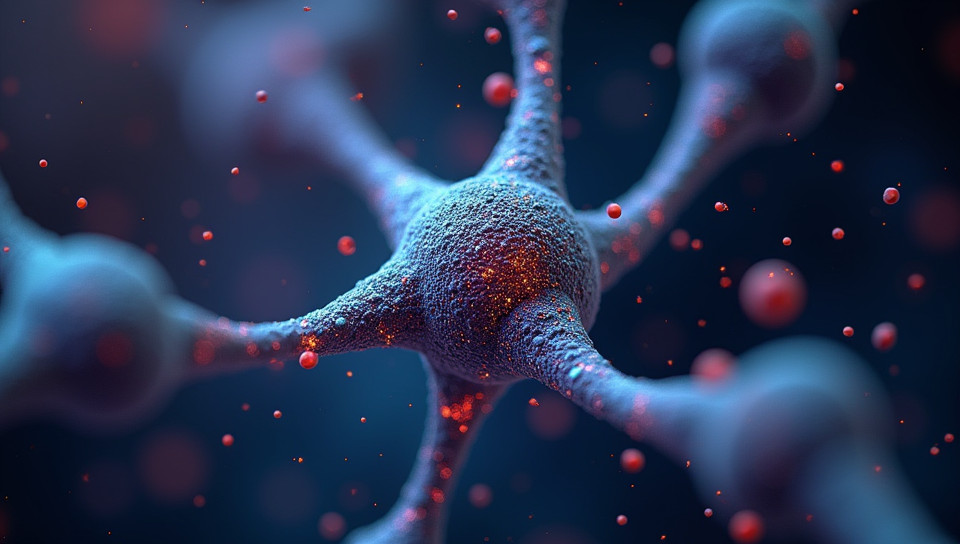
The Future of Chemistry: How Quantum Computers Revolutionize Molecular Simulations
Imagine being able to predict and understand the intricacies of molecular interactions with unprecedented accuracy, paving the way for groundbreaking discoveries in fields such as medicine, materials science, and environmental sustainability. This is now within reach thanks to the advent of quantum computers.
The Limitations of Classical Computing
Classical computers have long been the backbone of scientific research, but they face significant challenges when it comes to simulating complex molecular interactions. These interactions involve billions of particles interacting with each other in intricate ways, making it difficult for classical computers to accurately model and predict their behavior.
Quantum Leap: How Quantum Computers Tackle Molecular Simulations
Quantum computers, on the other hand, utilize the principles of quantum mechanics to perform calculations that are exponentially faster than those performed by classical computers. This is achieved through the use of quantum bits or qubits, which can exist in multiple states simultaneously, allowing for a vast increase in processing power.
- The ability to accurately simulate molecular interactions has far-reaching implications:
- Improved drug discovery and development
- Enhanced understanding of chemical reactions and their impact on the environment
- Development of new materials with unique properties
A New Era of Molecular Research
Quantum computers can tackle complex molecular simulations by leveraging quantum entanglement, superposition, and interference. These phenomena enable quantum computers to efficiently explore vast solution spaces and identify optimal solutions that classical computers cannot.
Conclusion
The arrival of quantum computers represents a significant breakthrough in the field of molecular research. By harnessing the power of quantum mechanics, scientists can now simulate complex molecular interactions with unprecedented accuracy, opening up new avenues for discovery and innovation. As we continue to push the boundaries of what is possible with quantum computing, we can expect to see profound impacts on various fields of science and beyond.
- Created by: Yǔtóng Suen
- Created at: Aug. 17, 2024, 12:33 a.m.
- ID: 7511