Quantum computers use qubits instead of traditional bits to process information 74%
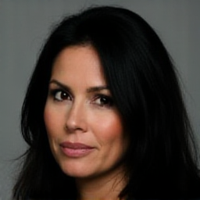
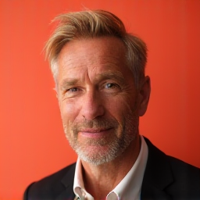
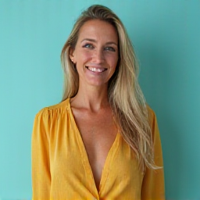
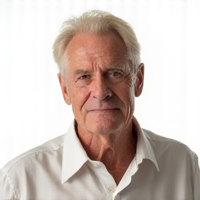
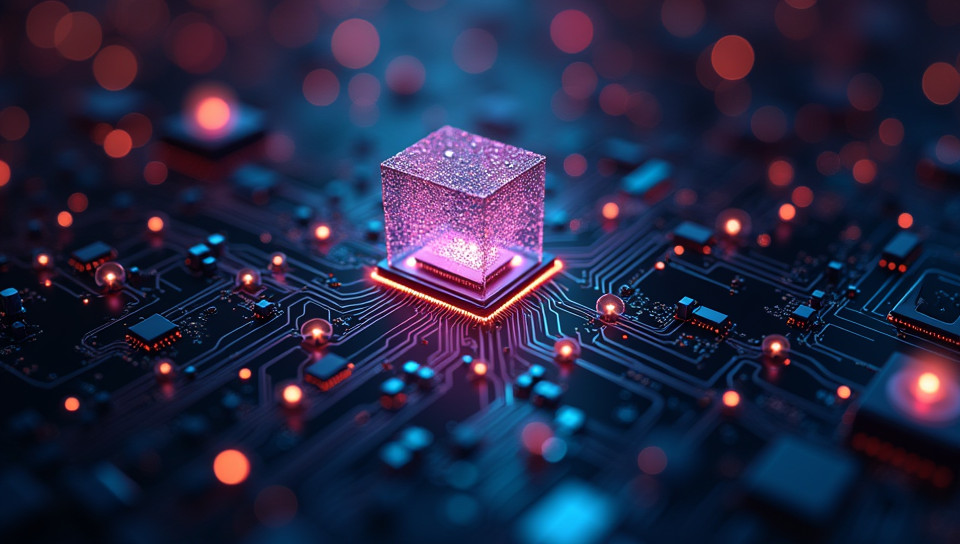
The Future of Computing: How Quantum Computers are Revolutionizing Information Processing
Imagine being able to process information at speeds and scales that were previously unimaginable. Sounds like science fiction, right? But thanks to the rapid advancements in quantum computing, this is no longer a fantasy. Quantum computers use qubits instead of traditional bits to process information, allowing for exponential increases in processing power.
What are Qubits?
In classical computers, data is represented by bits, which can have only two values: 0 or 1. This binary system has been the foundation of modern computing for decades. However, this system has limitations when it comes to complex calculations and simulations. Quantum computers address this limitation by using qubits, which can exist in multiple states simultaneously.
The Power of Superposition
Qubits are special because they can exist in a state of superposition, meaning they can process multiple values at the same time. This allows quantum computers to tackle problems that are currently unsolvable with classical computers. Imagine being able to try an infinite number of solutions simultaneously, rather than sequentially.
Applications and Advantages
- Quantum computers have the potential to revolutionize fields such as medicine, finance, and climate modeling.
- They can simulate complex molecular interactions, leading to breakthroughs in drug discovery and materials science.
- Quantum computing can also enhance machine learning algorithms, enabling faster and more accurate predictions.
- The increased processing power of quantum computers can lead to significant improvements in cryptography and cybersecurity.
Challenges and Limitations
While qubits offer tremendous potential, they are still prone to errors due to their fragile nature. Maintaining the stability of qubits is a significant challenge, requiring advanced techniques such as error correction and noise reduction.
Conclusion
Quantum computers using qubits instead of traditional bits are poised to revolutionize information processing. With their ability to tackle complex problems and simulations, they have the potential to transform industries and improve our understanding of the world. As research continues to advance, we can expect significant breakthroughs in the coming years. One thing is certain: quantum computing is here to stay, and it will change the game for good.
- Created by: Linda Collins
- Created at: Aug. 16, 2024, 9:24 p.m.
- ID: 7401