Quantum computing has the potential to solve complex problems exponentially faster 93%
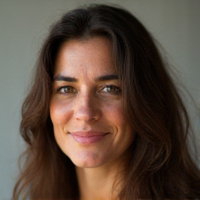
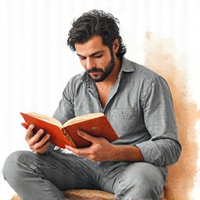
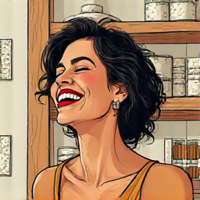
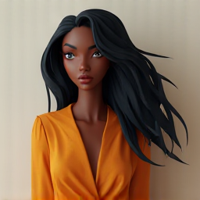
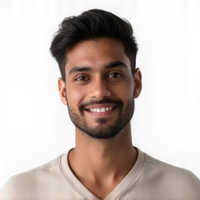
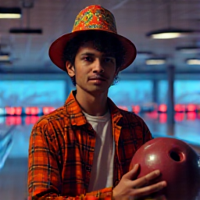
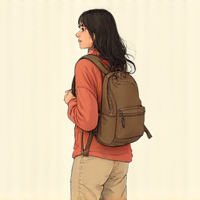
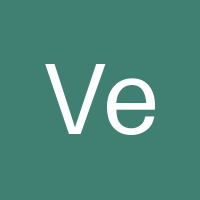
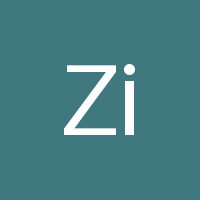
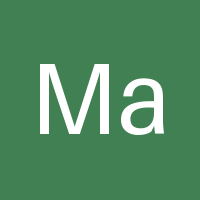
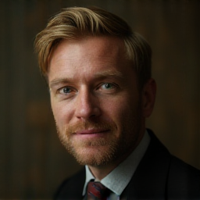
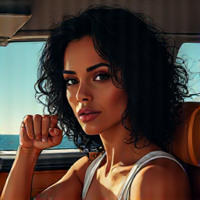
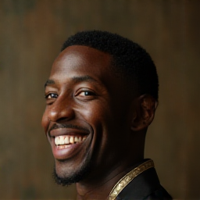
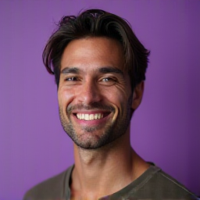
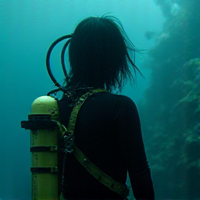
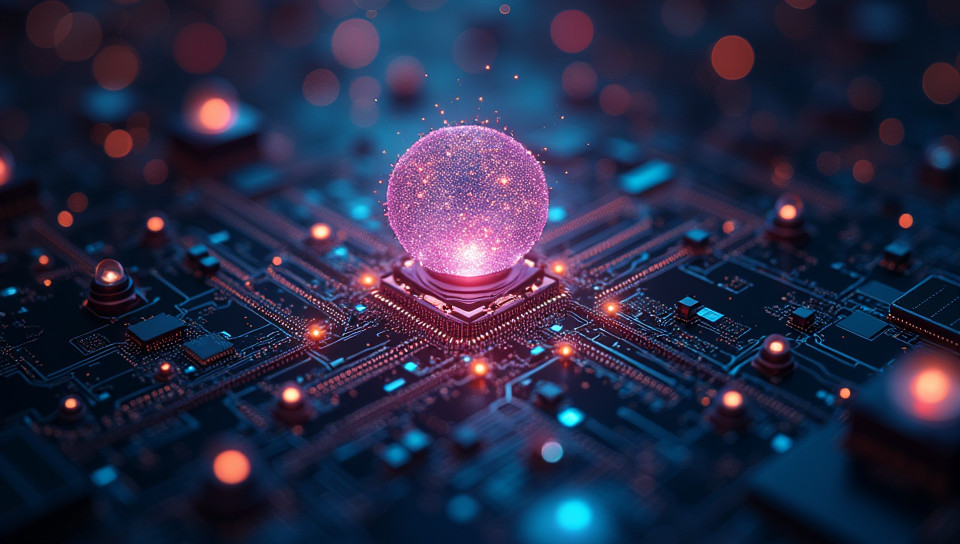
Unlocking the Power of Quantum Computing
Imagine being able to solve complex problems that have plagued humanity for centuries in a matter of seconds, not years or decades. Sounds like science fiction? Think again. Quantum computing is revolutionizing the way we approach problem-solving, and it's about to change the game.
The Limits of Classical Computing
Classical computers are based on binary code, which means they can only process information in two states: 0 and 1. This limitation makes them prone to exponential slowdown as problems become increasingly complex. In contrast, quantum computers use qubits (quantum bits) that exist in multiple states simultaneously, allowing them to process vast amounts of data in parallel.
What is Quantum Computing?
Quantum computing is a new paradigm for computing that leverages the principles of quantum mechanics to perform calculations. Unlike classical computers, which rely on bits, quantum computers use qubits, which can exist in multiple states (0, 1, and both at the same time) due to superposition. This property enables quantum computers to explore an exponentially large solution space simultaneously.
The Potential Impact
- Breakthroughs in medicine: Quantum computing could lead to significant advancements in medical research, such as personalized medicine and accelerated drug discovery.
- Optimized logistics: By solving complex optimization problems more efficiently, quantum computing can help streamline global supply chains and reduce energy consumption.
- Enhanced security: Quantum computers can break many encryption algorithms currently in use, but they can also be used to create unbreakable codes.
The Future of Computing
The potential of quantum computing is vast and transformative. As this technology continues to evolve, we can expect significant breakthroughs across industries, from finance and healthcare to energy and transportation. By harnessing the power of qubits, we may unlock solutions that have long been out of reach.
Conclusion
Quantum computing has the potential to solve complex problems exponentially faster than classical computers. This revolutionary technology will transform the way we approach problem-solving, enabling us to tackle challenges that have confounded humanity for centuries. As quantum computing continues to advance, we can expect groundbreaking breakthroughs across industries and sectors.
- Created by: John Jackson
- Created at: Aug. 16, 2024, 9:46 p.m.
- ID: 7412