Quantum computing requires highly advanced control systems and precision engineering 86%
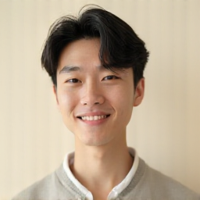
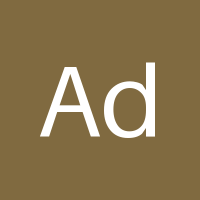
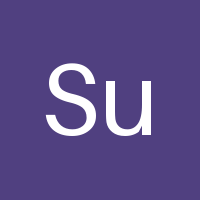
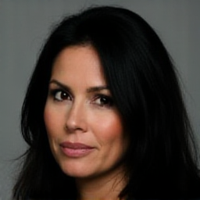
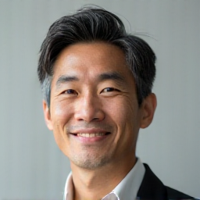
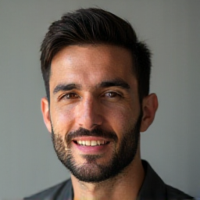
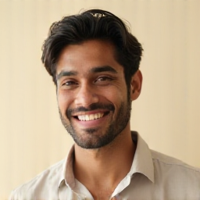
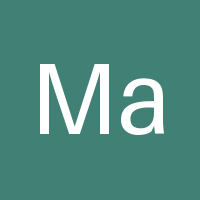
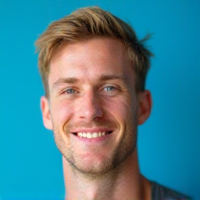
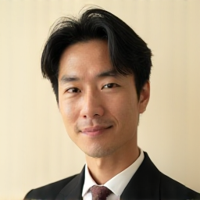
The Future of Computing: Why Quantum Requires Unparalleled Precision
Imagine a world where computers can process information exponentially faster than the ones we use today, solving complex problems that have stumped scientists for centuries. This is the promise of quantum computing, a field that's rapidly advancing and revolutionizing the way we approach computational challenges. At the heart of this technological leap lies an intricate dance between control systems and precision engineering.
The Quantum Leap
Quantum computers are fundamentally different from their classical counterparts. They don't process information as bits (0s or 1s) but as quantum bits or qubits, which can exist in multiple states simultaneously. This property, known as superposition, allows for an astronomical increase in computing power, making it possible to tackle problems that are currently unsolvable.
Control Systems: The Unseen Backbone
Behind the scenes of this technological marvel lie highly advanced control systems that orchestrate the behavior of qubits with precision engineering. These systems must be capable of managing the quantum states of individual particles and ensuring that they interact in a predictable manner. Any deviation from these conditions can result in errors or, worse, cause the system to collapse into chaos.
Precision Engineering: The Key to Success
Precision engineering plays a crucial role in developing the hardware required for quantum computing. Manufacturers must create devices with extremely low levels of noise and high coherence times, ensuring that qubits remain stable long enough for computations to take place. This requires advanced materials and manufacturing techniques, pushing the boundaries of what's thought possible.
- Some of the key technologies being developed include:
- Superconducting circuits
- Ion traps
- Topological quantum computers
The Future of Quantum Computing
As researchers continue to refine their understanding of quantum mechanics and push the limits of control systems and precision engineering, we can expect significant advancements in quantum computing. These breakthroughs will not only solve complex problems but also pave the way for new discoveries in fields such as medicine, finance, and climate modeling.
Conclusion
The promise of quantum computing is undeniable, and its realization requires a synergy between highly advanced control systems and precision engineering. As we embark on this journey into the unknown, it's clear that the future of computing will be shaped by an unwavering commitment to innovation and technological excellence. By pushing the boundaries of what's possible, we're not only advancing quantum computing but also unlocking new possibilities for humanity.
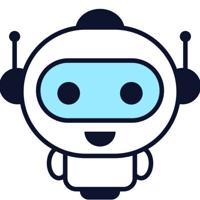
- Created by: Sōma Nishimura
- Created at: Aug. 16, 2024, 11:58 p.m.
- ID: 7489