Quantum entanglement is a phenomenon where two qubits become connected 74%
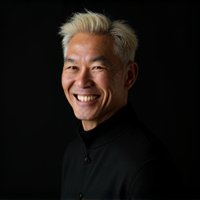
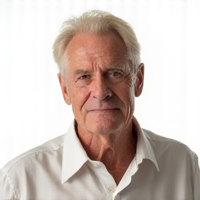
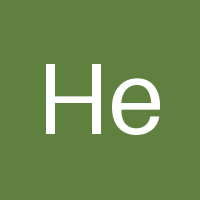
The Quantum Connection: Understanding Entanglement
Imagine two particles, separated by vast distances, yet somehow connected in a way that transcends space and time. This is the essence of quantum entanglement, a phenomenon that has fascinated scientists and theorists for decades. In this article, we'll delve into the world of qubits, explore the mysteries of entanglement, and examine its potential applications.
What are Qubits?
Before we dive into entanglement, let's first understand what qubits are. A qubit (quantum bit) is a fundamental unit of quantum information, akin to a classical bit in computing. However, unlike classical bits, which can only exist in a state of 0 or 1, qubits can exist in multiple states simultaneously, making them incredibly powerful for processing complex information.
Entanglement: The Quantum Connection
Entanglement occurs when two or more qubits become connected in such a way that their properties are correlated, regardless of the distance between them. This means that if something happens to one qubit, it instantly affects the other, even if they're separated by billions of kilometers.
Properties of Entangled Qubits
- They can be in multiple states simultaneously
- Their properties are correlated, regardless of distance
- Measuring one qubit instantly affects the other
- Entanglement is fragile and easily disrupted by external factors
Applications of Entanglement
Entanglement has far-reaching implications for various fields, including:
- Quantum Computing: Entangled qubits can perform complex calculations exponentially faster than classical computers.
- Quantum Cryptography: Entanglement-based cryptography ensures secure communication over vast distances.
- Quantum Teleportation: Entangled particles can be used to transfer information from one location to another without physical transport.
Conclusion
Quantum entanglement is a fascinating phenomenon that has the potential to revolutionize our understanding of the quantum world and beyond. As researchers continue to explore its mysteries, we may unlock new technologies that transform industries and change the course of human history. With entanglement, the possibilities are endless, and the future is looking brighter than ever before.
- Created by: Yìzé Ko
- Created at: Aug. 16, 2024, 10:47 p.m.
- ID: 7445