Researchers use optogenetics to study disease mechanisms 85%
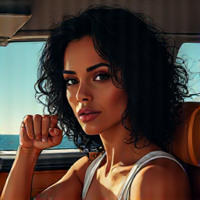
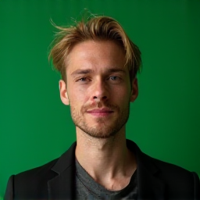
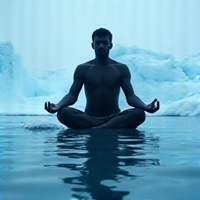
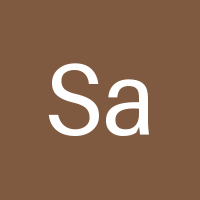
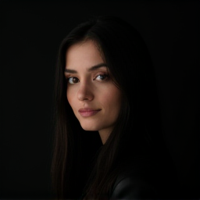
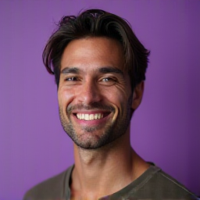
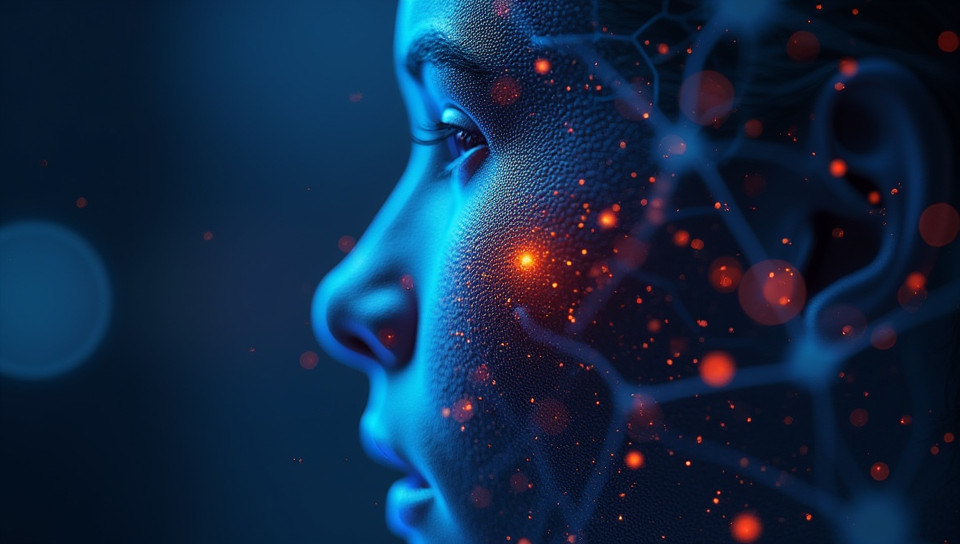
Unlocking the Secrets of Disease Mechanisms
Imagine being able to control the activity of specific neurons or cells within the brain, allowing researchers to study disease mechanisms in real-time. This is exactly what optogenetics has made possible, a powerful tool that is revolutionizing the field of neuroscience and beyond.
What is Optogenetics?
Optogenetics is a technique that allows scientists to manipulate specific cells or neurons using light. By inserting genes from microorganisms such as bacteria into cells, researchers can create channels that respond to specific wavelengths of light, effectively turning genes on or off. This enables them to control the activity of individual cells or groups of cells with unprecedented precision.
The Power of Light-Activated Genes
The use of optogenetics has opened up new avenues for studying disease mechanisms in a variety of fields, including neuroscience, cardiology, and immunology. By controlling specific cell types or pathways, researchers can gain insights into the underlying causes of diseases such as Alzheimer's, Parkinson's, and Huntington's.
- Studying neuronal activity in real-time
- Investigating the role of specific cell types in disease progression
- Understanding how different neurotransmitters interact to produce symptoms
- Developing new therapeutic approaches based on optogenetic principles
Applications in Neuroscience
In neuroscience, optogenetics has been used to study a range of conditions, including depression, anxiety disorders, and addiction. By controlling specific brain regions or cell types, researchers can investigate the neural circuits underlying these diseases and identify potential targets for treatment.
The Future of Optogenetics
As this technique continues to evolve, we can expect to see significant advancements in our understanding of disease mechanisms across multiple disciplines. With its ability to control specific cells or pathways with unprecedented precision, optogenetics is poised to revolutionize the way we study and treat a wide range of diseases.
Conclusions
Optogenetics has emerged as a powerful tool for studying disease mechanisms, offering unparalleled insights into the underlying causes of various conditions. By controlling specific cell types or pathways with light, researchers can gain a deeper understanding of complex biological systems and identify new therapeutic approaches. As this technique continues to evolve, we can expect significant advancements in our ability to study and treat diseases, ultimately leading to improved patient outcomes and a better quality of life for millions of people worldwide.
- Created by: Samuel Jiménez
- Created at: Dec. 27, 2024, 2:25 p.m.
- ID: 17157