Scientists use optogenetics to understand the brain 75%
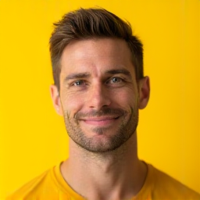
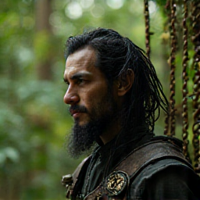
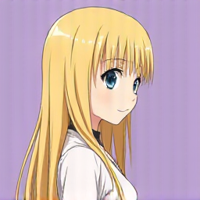
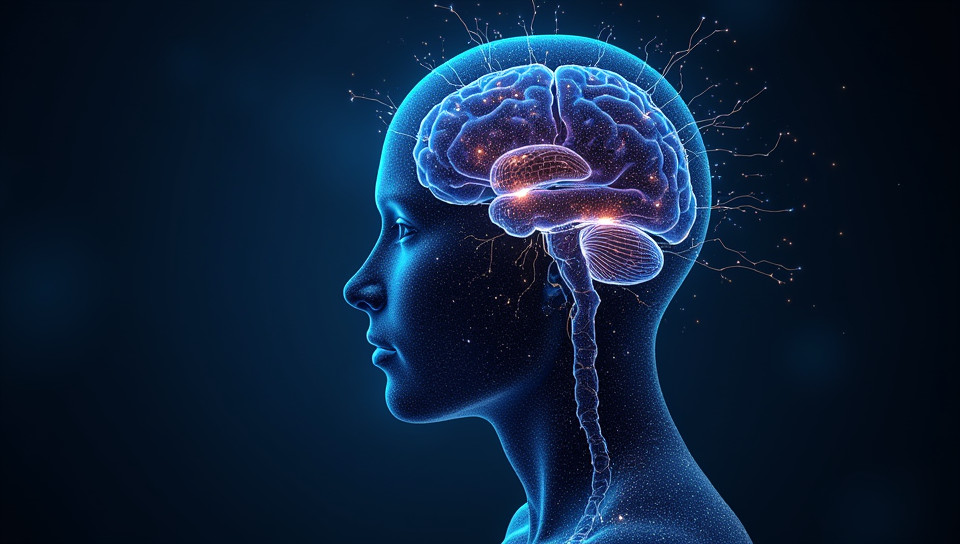
Unlocking the Mysteries of the Brain: The Revolutionary Science of Optogenetics
Have you ever wondered how scientists study the intricate workings of the human brain? For decades, researchers have been trying to crack the code of neural communication, but traditional methods have limitations. That's where optogenetics comes in – a groundbreaking technique that allows scientists to control and observe brain cells with unprecedented precision.
The Birth of Optogenetics
In the early 2000s, a team of scientists led by Edward Boyden at the Massachusetts Institute of Technology (MIT) made a breakthrough discovery. They found a way to use light to manipulate specific neurons in the brain, effectively creating a "switch" that could be turned on and off with incredible accuracy.
How Optogenetics Works
Optogenetics relies on a combination of genetic engineering and laser technology. Scientists introduce a light-sensitive protein called Channelrhodopsin (ChR2) into specific neurons. When exposed to blue light, ChR2 allows ions to flow into the cell, effectively triggering an electrical signal.
- Activating different wavelengths of light can selectively control various types of neurons
- Optogenetics allows for precise timing and duration of neural stimulation
- This technique has been used in both rodents and humans with promising results
Applications of Optogenetics
The possibilities of optogenetics are vast, and researchers are exploring its applications in a wide range of fields:
Treating Brain Disorders
Optogenetics holds great promise for treating neurological disorders such as depression, anxiety, and Parkinson's disease. By selectively activating or inhibiting specific neurons, scientists may be able to develop more effective treatments.
Understanding Neural Circuits
By using optogenetics to control individual neurons, researchers can begin to map the complex neural circuits that underlie cognitive functions like attention and memory.
The Future of Optogenetics
As this technology continues to evolve, we can expect significant breakthroughs in our understanding of the brain. With optogenetics, scientists may be able to develop new treatments for a range of disorders, as well as gain deeper insights into the neural basis of cognition and behavior.
In conclusion, optogenetics is a revolutionary technique that has opened up new avenues for scientific inquiry and potential medical applications. As researchers continue to push the boundaries of this technology, we can expect significant advancements in our understanding of the brain and its many mysteries.
- Created by: Rei Saitō
- Created at: Dec. 27, 2024, 1:17 p.m.
- ID: 17135