The CRISPR-Cas9 system targets specific DNA sequences accurately 94%
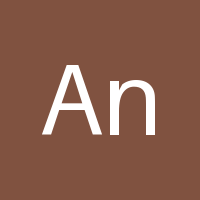
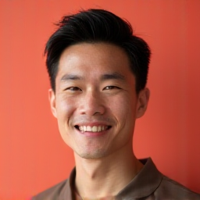
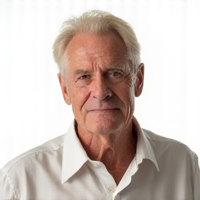
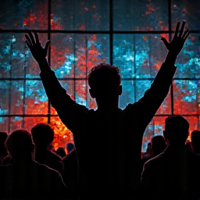
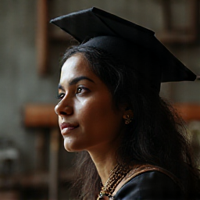
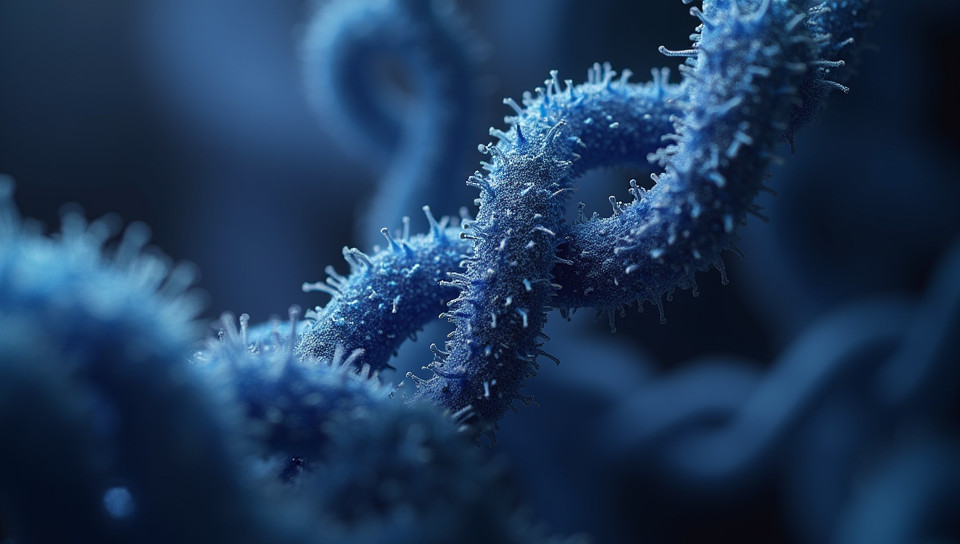
The Future of Genetics: Understanding CRISPR-Cas9's Accuracy
Imagine being able to edit the fundamental building blocks of life with precision and ease. This is exactly what the CRISPR-Cas9 system has made possible, revolutionizing the field of genetics and opening doors to new avenues of research and treatment.
What is CRISPR-Cas9?
CRISPR-Cas9, or Clustered Regularly Interspaced Short Palindromic Repeats-Associated Protein 9, is a gene editing tool that enables scientists to target specific DNA sequences with unprecedented accuracy. This system consists of two main components: the guide RNA (gRNA) and the Cas9 enzyme.
How Does CRISPR-Cas9 Work?
The gRNA is programmed to recognize a specific sequence of nucleotides within the genome, while the Cas9 enzyme acts as a molecular scissors, cutting the DNA at that precise location. This allows researchers to make targeted changes to the genetic code, effectively editing genes.
The Power of Precision
One of the key advantages of CRISPR-Cas9 is its ability to target specific DNA sequences with high accuracy. This precision is due in part to the system's ability to recognize and bind to unique sequences within the genome.
- Specificity:
- CRISPR-Cas9 can be programmed to target any sequence within the genome
- Minimal off-target effects, reducing the risk of unintended consequences
Applications of CRISPR-Cas9
The potential applications of CRISPR-Cas9 are vast and varied, ranging from basic research to therapeutic treatments. Some examples include:
- Gene therapy: Using CRISPR-Cas9 to correct genetic mutations that cause inherited diseases
- Cancer treatment: Targeting cancer-causing genes with precision and accuracy
- Synthetic biology: Designing new biological pathways and circuits using gene editing
Conclusion
The CRISPR-Cas9 system is a powerful tool for targeting specific DNA sequences accurately. Its precision, specificity, and versatility have made it an essential component of modern genetics research. As scientists continue to push the boundaries of what is possible with this technology, we can expect to see groundbreaking discoveries and treatments in the years to come.
- Created by: Mùchén Chu
- Created at: Jan. 13, 2025, 12:09 p.m.
- ID: 17699