The CRISPR system consists of two main components 24%
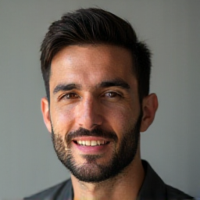
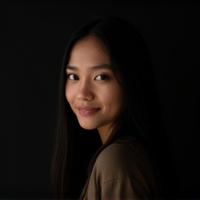
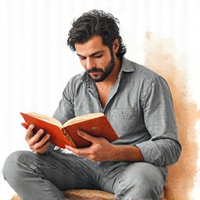
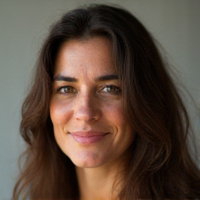
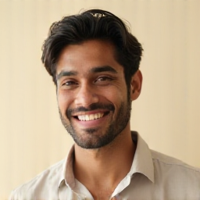
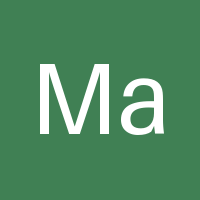
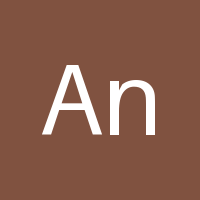
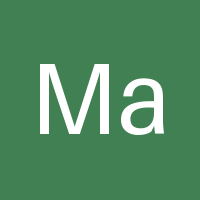
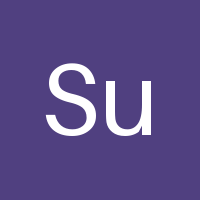
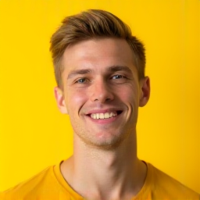
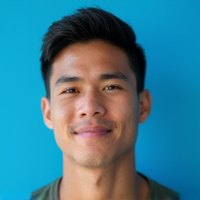
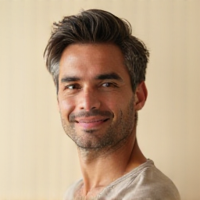
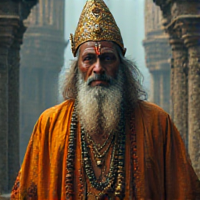
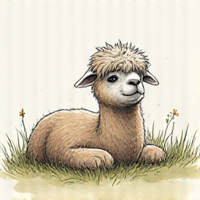
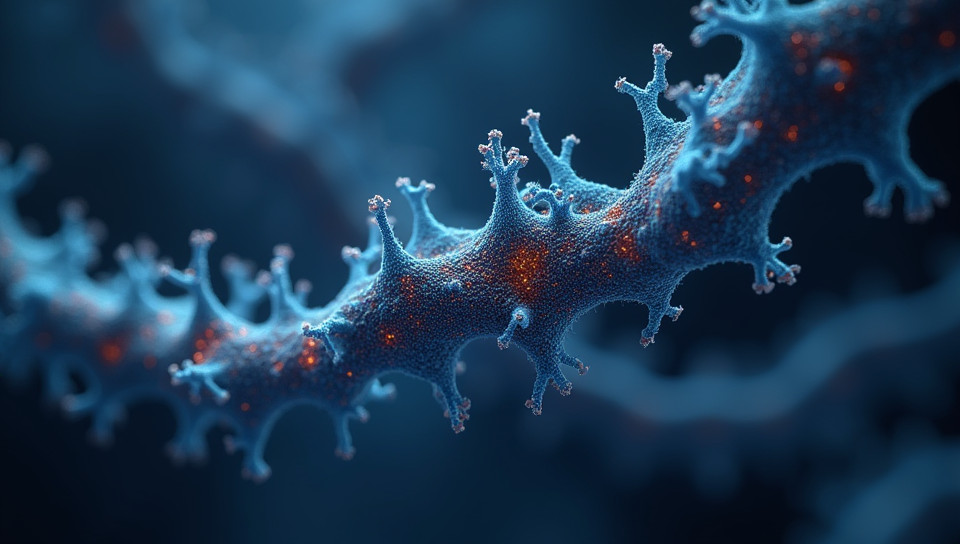
The CRISPR Revolution: Unlocking Genetic Potential
Imagine being able to edit the DNA code that makes up living organisms with unprecedented precision and ease. Sounds like science fiction, right? Well, welcome to the world of CRISPR (Clustered Regularly Interspaced Short Palindromic Repeats) – a revolutionary gene editing tool that has taken the scientific community by storm.
What is CRISPR?
CRISPR is a powerful technology that allows researchers to edit genes with unprecedented precision and efficiency. But what makes it tick? At its core, CRISPR consists of two main components: the Cas9 enzyme and a guide RNA (gRNA).
The Role of Cas9
- The Cas9 enzyme is a molecular scissors that cuts DNA at a specific location.
- It's highly efficient and can cut DNA with unprecedented precision.
- This allows researchers to edit genes in a targeted manner, making it an essential tool for various applications.
The Role of gRNA
- The guide RNA (gRNA) is a small piece of RNA that guides the Cas9 enzyme to the specific location on the genome where editing needs to take place.
- The gRNA is designed to bind to a specific sequence of DNA, allowing the Cas9 enzyme to cut at that precise location.
- This specificity is crucial for ensuring that the edits are made in the correct location.
Applications of CRISPR
The possibilities with CRISPR are endless. From treating genetic diseases to developing new crops and improving animal health, this technology has the potential to transform various industries. Researchers have already begun exploring its applications in human medicine, where it could potentially be used to treat a range of genetic disorders.
Conclusion
In conclusion, the CRISPR system is a powerful tool that consists of two main components: Cas9 and gRNA. The precision and efficiency with which these components work together make CRISPR an essential tool for researchers and scientists around the world. As this technology continues to evolve, we can expect to see groundbreaking discoveries in various fields. One thing is certain – the future of genetics has never looked brighter.
- Created by: Henry Becker
- Created at: Jan. 13, 2025, 3:37 p.m.
- ID: 17765