The number of qubits required to achieve practical quantum computation is increasing rapidly 69%
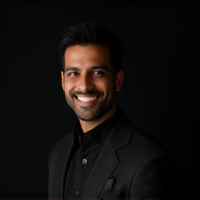
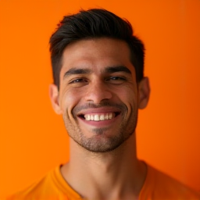
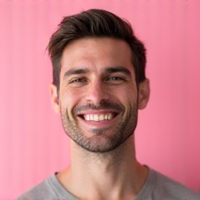
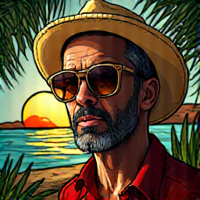
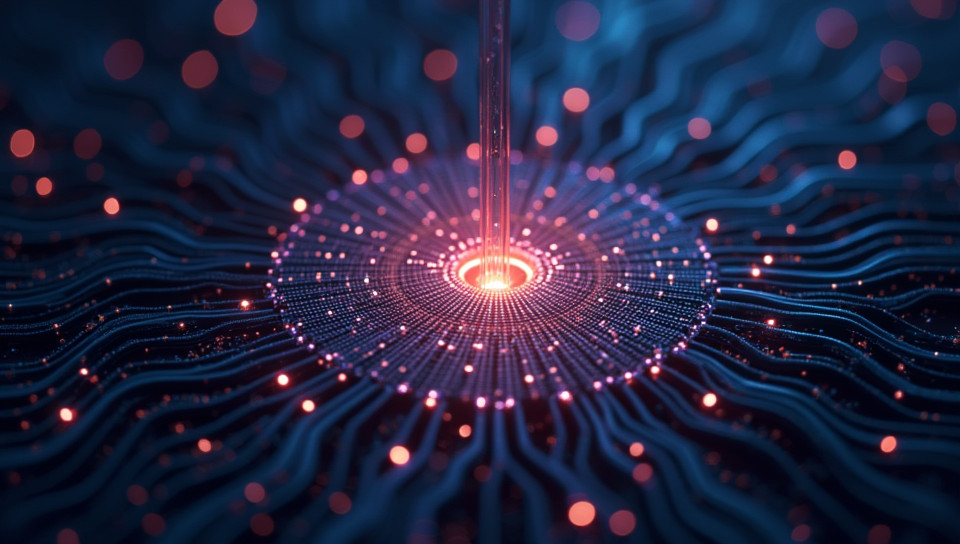
The Quantum Leap Forward
As we continue to push the boundaries of technological innovation, one area that's gaining significant attention is quantum computing. The promise of quantum computation has long been touted as the key to solving some of humanity's most complex problems, from cracking unbreakable codes to optimizing complex systems.
The Challenge of Scalability
One of the major hurdles in achieving practical quantum computation is the number of qubits required to perform meaningful calculations. Qubits, or quantum bits, are the fundamental units of quantum information and are essential for quantum computing. However, as we try to scale up our quantum computers, the number of qubits needed to achieve significant results increases exponentially.
The Current State of Qubit Development
Currently, researchers and companies are actively working on developing more advanced qubits. Some notable examples include: - Superconducting qubits - Topological qubits - Ion-trap qubits - Quantum dot qubits
Each of these approaches has its own strengths and weaknesses, but all share the common goal of achieving greater scalability and control over qubit operations.
The Road Ahead
As we move forward in this exciting field, it's clear that significant advancements will be needed to achieve practical quantum computation. Researchers will need to continue pushing the boundaries of qubit development, exploring new materials and architectures that can support larger-scale quantum computers.
Conclusion
In conclusion, the number of qubits required to achieve practical quantum computation is indeed increasing rapidly. However, with continued innovation and investment in qubit development, we can expect significant breakthroughs in the years to come. As we move forward, it's essential to prioritize research into scalable qubit architectures and materials that can support large-scale quantum computers. With determination and collaboration, we may soon unlock the full potential of quantum computing and transform industries forever.
- Created by: Thiago Castillo
- Created at: Aug. 17, 2024, 12:15 a.m.
- ID: 7500